Introduction
The bimolecular nucleophilic substitution, or SN2, is a common reaction mechanism in organic chemistry. This process involves a strong nucleophile that forms a new bond with an sp3 carbon atom through a backside attack, while the leaving group simultaneously departs from the reaction center. The reaction typically occurs at an aliphatic sp3 carbon center with a stable, electronegative leaving group (or one that can readily support a negative charge) attached. The nucleophile, denoted as Nu, forms a C–Nu bond concurrently with the breaking of the C–LG bond. This happens through a transition state where the reaction center is penta-substituted and approximately sp2-hybridised. The SN2 reaction can be viewed as an interaction between the highest occupied molecular orbital (HOMO) of the nucleophile and the lowest unoccupied molecular orbital (LUMO) of the substrate. The reaction only occurs when the nucleophile’s lone pair orbital donates electrons to the empty sigma* antibonding orbital between the central carbon and the leaving group. A p orbital forms at the reaction center during the transition from the reactants’ molecular orbitals to those of the products.
For optimal orbital overlap, the nucleophile attacks 180° relative to the leaving group, leading to the leaving group being pushed off the opposite side and the product formed with inversion of tetrahedral geometry at the central atom. If the substrate undergoing the SN2 reaction has a chiral center, then inversion of configuration may occur.
Reaction
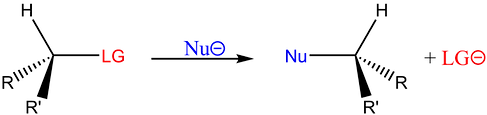
-
1-step reaction involving substrate and nucleophile
-
The reaction involves a “back-side attack.”
-
There is always an inversion of stereochemistry
Mechanism

-
The nucleophile’s highest occupied molecular orbital (HOMO) donates an electron pair to the C-LG lowest unoccupied molecular orbital (LUMO) to form the Nu-C bond and to break the C-LG bond.
Electrophile
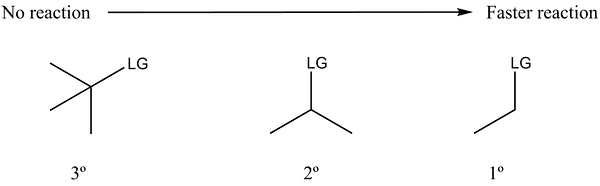
The electrophile's structure plays a crucial role in determining the rate of an SN2 reaction. For an SN2 reaction to proceed rapidly, the nucleophile must have easy access to the sigma antibonding orbital between the central carbon and the leaving group. The reaction rate of SN2 is faster with substrates that have less steric hindrance at the central carbon, meaning those that do not have bulky substituents nearby. Methyl and primary substrates are the most reactive, followed by secondary substrates. Tertiary substrates, due to their greater steric hindrance, do not typically undergo SN2 reactions, as the SN1 reaction pathway is often favored in these cases.
Nucleophile
Just as the substrate, the nucleophile’s strength is also affected by steric hindrance. The strength of a nucleophile is also influenced by its charge and electronegativity: the more negative the charge and the lower the electronegativity, the stronger the nucleophile. For example, the hydroxide ion (OH−) is a stronger nucleophile than water, and iodide (I−) is a stronger nucleophile than bromide (Br−) in polar protic solvents.
In a polar aprotic solvent, nucleophilicity increases up a column of the periodic table because there is no hydrogen bonding between the solvent and nucleophile. In this case, nucleophilicity aligns closely with basicity levels. Therefore, iodide, being less basic, is not as strong a nucleophile as bromide.
Summary:
-
A strong or negatively charged nucleophile tends to favor the SN2 pathway for nucleophilic substitution.
Effect of Charge on Nucleophilicity
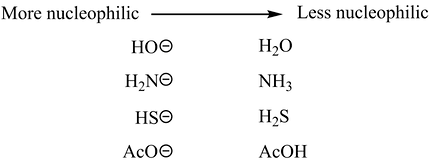
Effect of Electronegativity on Nucleophilicity
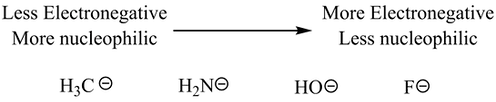
Effect of Steric Bulk on Nucleophilicity

Leaving Groups
The rate of SN2 reactions is significantly influenced by the 'quality' of the leaving groups attached to the substrate. These groups are characterized by their ability to accommodate the electron density that results from the breaking of their bond with the carbon atom. The effectiveness of a leaving group often correlates with the pKa value of its conjugate acid; a lower pKa indicates a faster displacement of the leaving group.
Neutral leaving groups, such as water, alcohols (R−OH), and amines (R−NH2), are beneficial due to their positive charge when connected to the carbon center before the nucleophilic attack. Halides like chloride, bromide, and iodide (excluding fluoride) are also considered effective anionic leaving groups, as their electronegativity helps in stabilizing the extra electron density. The exception of fluoride is due to its strong bond with carbon.
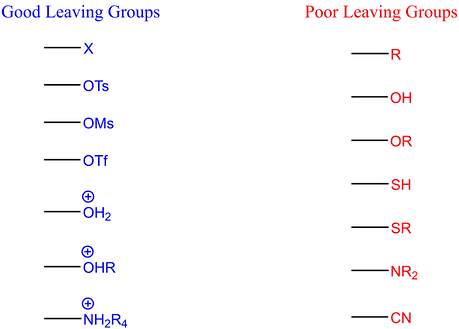
Solvents
The solvent plays a significant role in determining the reaction rate as it can either encase or leave the nucleophile exposed, thereby facilitating or obstructing its approach to the carbon atom. Polar aprotic solvents are more conducive to SN2 than polar protic solvents. This is because polar protic solvents form hydrogen bonds with the nucleophile, which hinders its ability to attack the carbon atom attached to the leaving group. A polar aprotic solvent with a low dielectric constant or a hindered dipole end is more favorable for the SN2 nucleophilic substitution reaction. Furthermore, the solvation process significantly impacts the inherent strength of the nucleophile. While polar protic solvents form strong interactions with the nucleophile, leading to a reduction in nucleophilic strength, polar aprotic solvents interact minimally, thereby preserving the nucleophile’s potency.
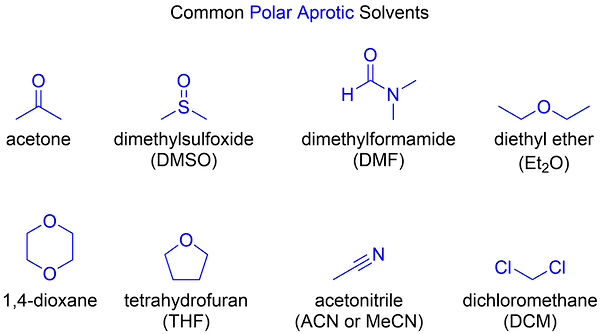
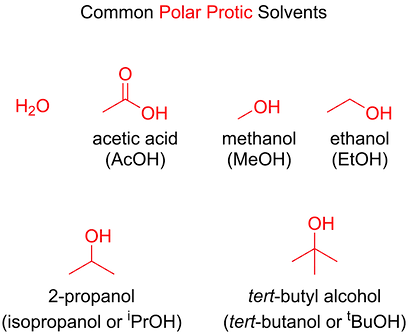
Nucleophilicity Trend in Polar Aprotic Solvents

-
Polar aprotic solvents do not hydrogen-bond to nucleophiles, allowing them more freedom to attack electrophiles in solution
-
Nucleophilicity correlates well with basicity (F- is more basic than I-)
Nucleophilicity Trend in Polar Aprotic Solvents

-
A polar protic solvent can create a “shell” around the nucleophile through hydrogen-bonding, lowering its ability to attack an electrophile
-
F- is a stronger hydrogen bond acceptor than I-
-
In a polar protic solvent, the lone pair of iodide is more free than the lone pair of fluoride, and can be more easily donated to an electrophile, hence making iodide a better nucleophile
-
Keywords
bimolecular substitution | sn2 | nucleophile | leaving group | electrophile | protic | aprotic